Riley Lab Research Projects
The inner ear is derived from a simple epithelial thickening, the otic placode. The otic placode goes on the produce a fluid filled otic vesicle, which in turn undergoes extensive growth and morphogeneisis to produce the highly complex structure of the adult inner ear.
Understanding the mechanisms that establish the otic placode has been the focus of numerous studies for nearly half a century.
dlx3b (PPE)
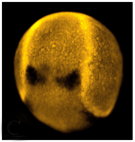
wnt8 (hindbrain)
The otic placode is one of a series of cranial placodes that form around the head in all vertebrate embryos. Placodes later establish key parts of the paired sensory structures of the head. All placodes arise from a common domain of “preplacodal extoderm” (PPE) bordering the anterior neural plate. We are investigating the molecular and cellular mechanisms that regulate formation of the PPE during gastrulation.
Diverse placodes arise from different parts of the PPE in response to various local signals. We discovered that Fibroblast Growth Factor (Fgf) from the hindbrain triggers otic differentiation in adjacent PPE cells. We are studying transcription factors (Pax2, Pax8, Sox3) that control these early steps. We are also investigating subsequent steps whereby otic cells converge to form a thickened placode. Convergence of otic cells is regulated by Integrins, transmembrane proteins that facilitate cell adshesion, directed cell migration, and cell signalling.
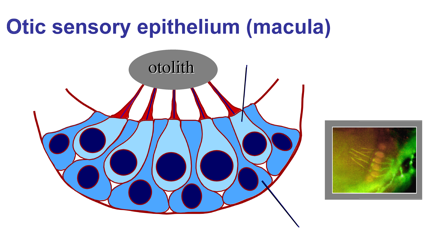
Hearing and balance are mediated by sensory hair cells, named for mechano-sensory cilia projecting from their apical surfaces into the interior of the ear. Sound waves or force from acceleration can deflect cilia, opening mechanically gated ion channels that activate hair cells. In some regions of the inner ear, the tips of hair cell cilia are attached to dense crystals (otoliths) that enhance mechano-transduction. Hair cells are interspersed with support cells, which are required for hair cell survival and also play a vital role in hair cell regeneration (see below).
Sensory Development: We are investigating genes that regulate development of sensory epithelia. Hair cells and support cells are derived from a common pool of precursors (a sensory equivalence group). The transcription factor Atoh1 is known to initiate hair cell differentiation, but we recently found that it also plays an earlier role in establishing the entire equivalence group. Loss of Atoh1 prevents formation of both hair cells and support cells. Additionally, over-expression of Atoh1 using a heat shock-inducible transgene (hsp70-atoh1) leads to dramatic over-production of sensory epithelia containing both support cells and hair cells. The above photos were taken of live embryos in a genetic background in which all hair cells express GFP fluorescence. Excess hair cells can be clearly seen in a hsp70-atoh1 transgenic embryo. Excess support cells are also present but are not visible under these conditions. Note that not all regions of the otic vesicle respond by producing sensory epithelia. We are studying how Atoh1 interacts with other genes to promote sensory competence (the ability to produce sensory epithelia).
Regeneration: Hair cells are sensitive to loud noise or other trauma that can lead to their death. Mammals (including humans) lack the ability to replace lost hair cells, but zebrafish display a robust capacity for hair cell regeneration. To study this process, we kill hair cells using a laser beam and monitor the response over time. As seen here, regeneration occurs within 24 hours after ablation. As hair cells die, nearby support cells undergo “transdifferentiation”. That is, they directly transform into new hair cells.
Identifying genes that regulate regeneration in zebrafish could provide insights for restoring hair cells in humans. We found that the transcription factor Sox2 is required for regeneration. Sox2 is expressed in support cells in both humans and zebrafish, but in mammals the level of expression is much lower. We hypothesize that Sox2 maintains “pluripotency” in support cells, facilitating regeneration.
Hearing (auditory function) and balance (vestibular function) develop in distinct regions of the inner ear, a process that is still poorly understood. These functions are already partially established in zebrafish embryos at a very early stage when the inner ear comprises an otic vesicle with only two sensory epithelia, the utricular and saccular maculae. These maculae communicate with auditory and vestibular processing centers in the brain through distinct sets of otic neurons. Genes preferentially expressed in one macula or the other (or their innervating neurons) potentially regulate functional specialization. We have identified several genes that specifically affect the utricular macula, loss of which destroys vestibular function and is invariably lethal. For example, we recently found that the transcription factor Pax5 is induced in the utricular macula by Fgf3 from the hindbrain. Loss of Pax5 leads to sporadic death of hair cells in the utricular macula and impairs vestibular function, while the saccular macula and hearing remain normal. We do not yet know why utricular hair cells alone require Pax5 for survival.
We are also trying to identify genes expressed only in the saccular macula that might specifically regulate hearing. Additionally, we are trying to establish how otic neurons faithfully “wire” these maculae to their respective processing centers in the brain, and how different functional classes of otic neurons are regulated during development (see below).
Neurons that innervate hair cells exist in a large cluster known as the SAG (stato-acoustic ganglion). SAG neuroblasts initially form in the otic vesicle (specification) near the utricular macula. They soon delaminate from the otic vesicle and undergo a protracted phase of migration and cell division (transit-amplification).
Finally SAG cells differentiate into mature neurons and extend processes to interconnect hair cells with appropriate sensory processing centers in the brain. We are attempting to identify genes controlling each step during SAG development. We recently found that mature SAG neurons secrete Fgf, which acts as a feedback inhibitor of further SAG development. As mature SAG neurons accumulate, levels of Fgf increase to terminate further specification and suspend transit-amplification. This prevents over-production of mature neurons and maintains a pool of quiescent stem cells/progenitors. Ablation of mature SAG neurons disrupts feedback inhibition, which reactivates transit-amplification and thereby regenerates the mature SAG population.
A genetic screen: We are currently screening for mutations in a transgenic background in which mature SAG neurons express GFP (isl2b:GFP). The transgene allows detection of mutations that change the number or distribution of SAG neurons. We have so far found two mutations with specific defects: One shows a specific deficiency of vestibular neurons; the other shows a specific deficiency of auditory neurons. We are mapping the mutations to identify the affected genes. We believe that further analysis of these genes will shed light on the mechanisms that distinguish auditory from vestibular neurons. We anticipate that further screening that will identify other genes that specifically control different steps in the SAG developmental pathway.